This post was originally published on this site
Until recently, gravitational waves could have been a figment of Einstein’s imagination. Before they were detected, these ripples in spacetime existed only in the physicist’s general theory of relativity, as far as scientists knew.
Now, researchers have not one but two ways to detect the waves. And they’re on the hunt for more. The study of gravitational waves is booming, says astrophysicist Karan Jani of Vanderbilt University in Nashville. “This is just remarkable. No field I can think of in fundamental physics has seen progress this fast.”
Just as light comes in a spectrum, or a variety of wavelengths, so do gravitational waves. Different wavelengths point to different types of cosmic origins and require different flavors of detectors.
Gravitational waves with wavelengths of a few thousand kilometers — like those detected by LIGO in the United States and its partners Virgo in Italy and KAGRA in Japan — come mostly from merging pairs of black holes 10 or so times the mass of the sun, or from collisions of dense cosmic nuggets called neutron stars (SN: 2/11/16). These detectors could also spot waves from certain types of supernovas — exploding stars — and from rapidly rotating neutron stars called pulsars (SN: 5/6/19).
In contrast, immense ripples that span light-years are thought to be created by orbiting pairs of whopper black holes with masses billions of times that of the sun. In June, scientists reported the first strong evidence for these types of waves by turning the entire galaxy into a detector, watching how the waves tweaked the timing of regular blinks from pulsars scattered throughout the Milky Way (SN: 6/28/23).
With the equivalent of both small ripples and major tsunamis in hand, physicists now hope to plunge into a vast, cosmic ocean of gravitational waves of all sorts of sizes. These ripples could reveal new details about the secret lives of exotic objects such as black holes and unknown facets of the cosmos.
“There’s still a lot of gaps in our coverage of the gravitational wave spectrum,” says physicist Jason Hogan of Stanford University. But it makes sense to cover all the bases, he says. “Who knows what else we might find?”
This quest to capture the full complement of the universe’s gravitational waves could take observatories out into deep space or the moon, to the atomic realm and elsewhere.
Here’s a sampling of some of the frontiers scientists are eyeing in search of new types of waves.
Go to deep space
The Laser Interferometer Space Antenna, or LISA, sounds implausible at first. A trio of spacecraft, arranged in a triangle with 2.5-million-kilometer sides, would beam lasers to one another while cartwheeling in an orbit around the sun. But the European Space Agency mission, planned for the mid-2030s, is no mere fantasy (SN: 6/20/17). It is many scientists’ best hope for breaking into new realms of gravitational waves.
“LISA is a mind-blowing experiment,” says theoretical physicist Diego Blas Temiño of Universitat Autònoma de Barcelona and Institut de Física d’Altes Energies.
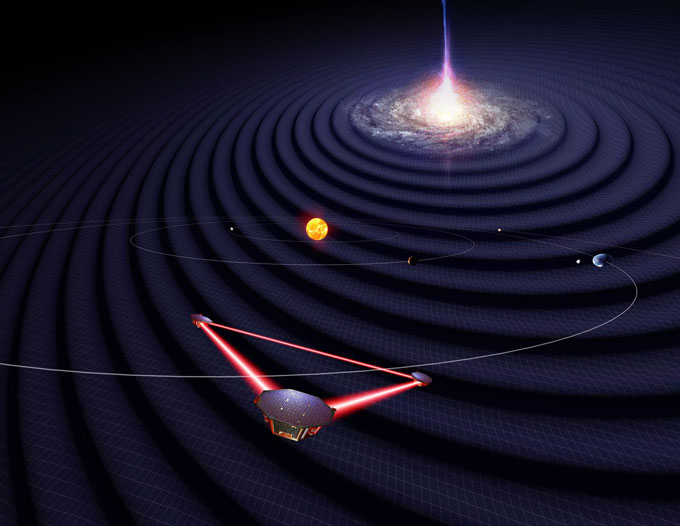
As a gravitational wave passes by, LISA would detect the stretching and squeezing of the sides of the triangle, based on how the laser beams interfere with each other at the triangle’s corners. A proof-of-concept experiment with a single spacecraft, LISA Pathfinder, flew in 2015 and demonstrated the feasibility of the technique (SN: 6/7/16).
Generally, to catch longer wavelengths of gravitational waves, you need a bigger detector. LISA would let scientists see wavelengths millions of kilometers long. That means LISA could detect orbiting black holes that would be enormous, but moderately so — millions of times the mass of the sun instead of billions.
Go to the moon
With NASA’s Artemis program aiming at a return to the moon, scientists are looking to Earth’s neighbor for inspiration (SN: 11/16/22). A proposed experiment called the Laser Interferometer Lunar Antenna, or LILA, would put a gravitational wave detector on the moon.
Without the jostling of human activity and other earthly jitters, gravitational waves should be easier to pick out on the moon. “It’s almost like a spiritual quietness,” Jani says. “If you want to listen to the sounds of the universe, these is no place better in the solar system than our moon.”
Like LISA, LILA would have three stations beaming lasers in a triangle, though the sides of this one would be about 10 kilometers long. It could catch wavelengths tens or hundreds of thousands of kilometers long. That would fill in a gap between the wavelengths measured by the space-based LISA and the Earth-based LIGO.
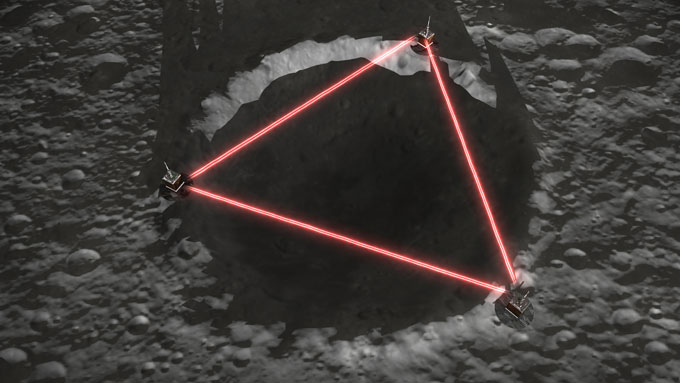
Because orbiting objects like black holes speed up as they get closer to merging, over time they emit gravitational waves with shorter and shorter wavelengths. That means LILA could watch black holes close in on one another during the weeks before they merge, giving scientists a heads-up that a collision is about to go down. Then, once the wavelengths get short enough, earthly observatories like LIGO would pick up the signal, catching the moment of impact.
A different moon-based option would use lunar laser ranging — a technique by which scientists measure the distance from Earth to the moon with lasers, thanks to reflectors placed on the moon’s surface during previous moon landings.
The method could detect waves jostling the Earth and the moon, with wavelengths in between those seen by pulsar timing methods and LISA, Blas Temiño and a colleague reported in Physical Review D in 2022. But that technique would require improved reflectors on the moon — another reason to go back.
Go atomic
LISA, LIGO and other laser observatories measure the stretching and squeezing of gravitational waves by monitoring how laser beams interfere after traversing their detectors’ long arms. But a proposed technique goes a different route.
Rather than looking for slight changes in the lengths of detector arms as gravitational waves pass, this new technique keeps an eye on the distance between two clouds of atoms. The quantum properties of atoms mean that they act like waves that can interfere with themselves. If a gravitational wave passes through, it changes the distance between the atom clouds. Scientists can tease out that change in distance based on that quantum interference.
The technique could reveal gravitational waves with wavelengths between those detectable by LIGO and LISA, Hogan says. He’s part of an effort to build a prototype detector, called MAGIS-100, at Fermilab in Batavia, Ill.
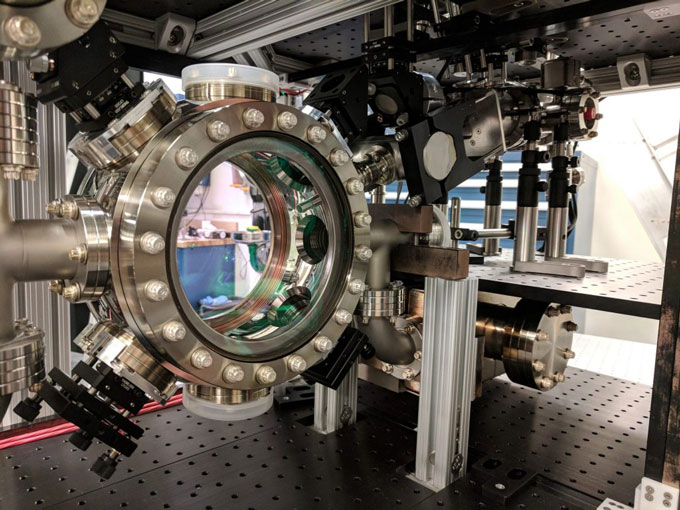
Atom interferometers have never been used to measure gravitational waves, though they can sense Earth’s gravity and test fundamental physics rules (SN: 2/28/22; SN: 10/28/20). The idea is “totally futuristic,” Blas Temiño says.
Go back in time
Another effort aims to pinpoint gravitational waves from the earliest moments of the universe. Such waves would have been produced during inflation, the moments after the Big Bang when the universe ballooned in size. These waves would have longer wavelengths than ever seen before — as long as 1021 kilometers, or 1 sextillion kilometers.
But the hunt got off to a false start in 2014, when scientists with the BICEP2 experiment proclaimed the detection of gravitational waves imprinted in swirling patterns on the oldest light in the universe, the cosmic microwave background, or CMB. The claim was later overturned (SN: 1/30/15).
An effort called CMB-Stage 4 will continue the search, with plans for multiple new telescopes that would scour the universe’s oldest light for signs of the waves — this time, hopefully, without any missteps.
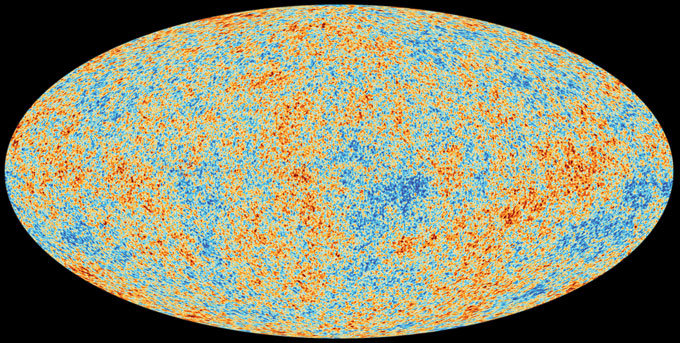
Go for the unknown
For most types of gravitational waves that scientists have set their sights on, they know a bit about what to expect. Known objects — like black holes or neutron stars — can create those waves.
But for gravitational waves with the shortest wavelengths, perhaps just centimeters long, “the story is different,” says theoretical physicist Valerie Domcke of CERN near Geneva. “We have no known source … that would actually give us [these] gravitational waves of a large enough amplitude that we could realistically detect them.”
Still, physicists want to check if the tiny waves are out there. These ripples could be produced by violent events early in the universe’s history such as phase transitions, in which the cosmos converts from one state to another, akin to water condensing from steam into liquid. Another possibility is tiny, primordial black holes, too small to be formed by standard means, which might have been born in the early universe. Physics in these regimes is so poorly understood, “even looking for [gravitational waves] and not finding them would tell us something,” Domcke says.
These gravitational waves are so mysterious that their detection techniques are also up in the air. But the wavelengths are small enough that they could be seen with high-precision, laboratory-scale experiments, rather than enormous detectors.
Scientists might even be able to repurpose data from experiments designed with other goals in mind. When gravitational waves encounter electromagnetic fields, the ripples can behave in ways similar to hypothetical subatomic particles called axions (SN: 3/17/22). So experiments searching for those particles might also reveal mini gravitational waves.
A new view
Catching gravitational waves is like paddling against the tide: tough going, but worth it for the scenic views. “Gravitational waves are really, really hard to detect,” Hogan says. It took decades of work before LIGO spotted its first swells, and the same is true of the pulsar timing technique. But astronomers immediately began reaping the rewards. “It’s a whole new view of the universe,” Hogan says.
Already, gravitational waves have helped confirm Einstein’s general theory of relativity, discover a new class of black holes of moderately sized masses and unmask the fireworks that happen when two ultradense objects called neutron stars collide (SN: 2/11/16; SN: 9/2/20; SN: 10/16/17).
And it’s still early days for gravitational wave detection. Scientists can only guess at what future detectors will expose. “There’s way more to discover,” Hogan says. “It’s bound to be interesting.”
.image-mobile {
display: none;
}
@media (max-width: 400px) {
.image-mobile {
display: block;
}
.image-desktop {
display: none;
}
}