At Bumpass Hell in California’s Lassen Volcanic National Park, the ground is literally boiling, and the aroma of rotten eggs fills the air. Gas bubbles rise through puddles of mud, producing goopy popping sounds. Jets of scorching-hot steam blast from vents in the earth. The fearsome site was named for the cowboy Kendall Bumpass, who in 1865 got too close and stepped through the thin crust. Boiling, acidic water burned his leg so badly that it had to be amputated.
Some scientists contend that life on our planet arose in such seemingly inhospitable conditions. Long before creatures roamed the Earth, hot springs like Bumpass Hell may have promoted chemical reactions that linked together simple molecules in a first step toward complexity. Other scientists, however, place the starting point for Earth’s life underwater, at the deep hydrothermal vents where heated, mineral-rich water billows from cracks in the ocean floor.
As researchers study and debate where and how life on Earth first ignited, their findings offer an important bonus. Understanding the origins of life on this planet could offer hints about where to search for life elsewhere, says Natalie Batalha, an astrophysicist at the University of California, Santa Cruz. “It has very significant implications for the future of space exploration.” Chemist Wenonah Vercoutere agrees. “The rules of physics are the same throughout the whole universe,” says Vercoutere, of NASA’s Ames Research Center in Moffett Field, Calif. “So what is there to say that the rules of biology do not also carry through and are in place and active in the whole universe?”
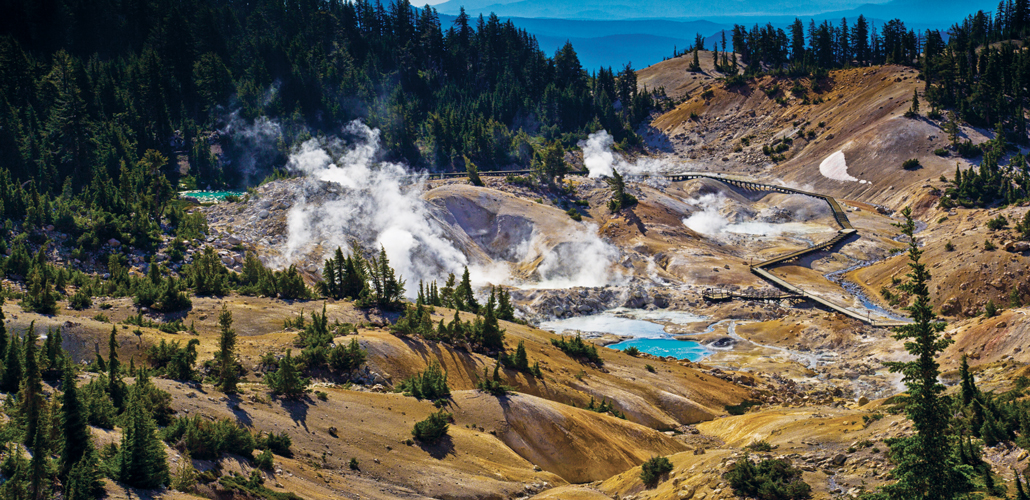
Lure of the land
At its biochemical core, the recipe for life relies on only a few ingredients: chemical elements, water or other media where chemical reactions can occur and an energy source to power those reactions. On Earth, all of those ingredients exist at terrestrial hot springs, home to some hardy creatures. Great Boiling Spring in Nevada, for example, is a scalding 77° Celsius, yet microbes manage to eke out an existence in water near the spring’s clay banks, researchers reported in 2016 in Nature Communications. Such conditions may reflect what it was like on early Earth, so these life-forms are most likely “related to some of the organisms that were originally on this planet,” says Jennifer Pett-Ridge, a microbial ecologist at Lawrence Livermore National Laboratory in California.
Microorganisms at hot springs can form communities called microbial mats. Made up of layers of microbes, mats have been found in geothermal areas all over the world, including in Yellowstone National Park, the Garga hot spring in southern Russia and Lassen — home to Bumpass Hell.
Over time, microbial mats can form into stromatolites, structures of microbes and minerals that have accumulated on top of one another; the layered appearance of a stromatolite reflects the passage of time, like a tree’s growth rings. Researchers found evidence of stromatolites in the Dresser Formation, a 3.5-billion–year-old rock feature in the Western Australia outback, along with evidence of hot spring mineral deposits, describing the findings in 2017 in Nature Communications. These findings, plus other signs of past microbes, led the team to suggest that some of the earliest life on Earth flourished in a hot spring environment.
David Deamer, a biophysicist at UC Santa Cruz, has spent four and a half decades exploring how life on our planet may have begun. He started out studying lipids, oily molecules that make up the membranes surrounding cells. Deamer, a big proponent of hot springs as the source of life’s start, has shown that conditions at terrestrial hot springs can produce bubblelike vesicles, with an outer layer made up of lipids. Such structures may have been the ancestral precursors of modern-day cells (SN: 7/3/10, p. 22).
Bruce Damer, an astrobiologist at UC Santa Cruz who brings a computer science approach to questions about the origins of life, worked with Deamer to test whether conditions at hot springs could drive condensation reactions, which join two molecules into one larger composite.
When water splashes out of a hot spring and evaporates, molecules that were in the liquid could undergo condensation reactions and link up. A subsequent splash would add more molecules that could undergo additional condensation reactions as liquid dries again. Repeated rounds of wetting and drying could produce chains of molecules.
In 2018, Damer set up shop at an active geothermal area in New Zealand, named along the usual theme — Hells Gate — to test that hypothesis. He prepared vials with ingredients needed to assemble strands of RNA, a nucleic acid that acts as a messenger during protein synthesis and may have catalyzed chemical reactions involved in the origins of life on early Earth (SN: 4/10/04, p. 232). The concoction included two of the four RNA building blocks — the nucleotides that link together to form RNA chains.
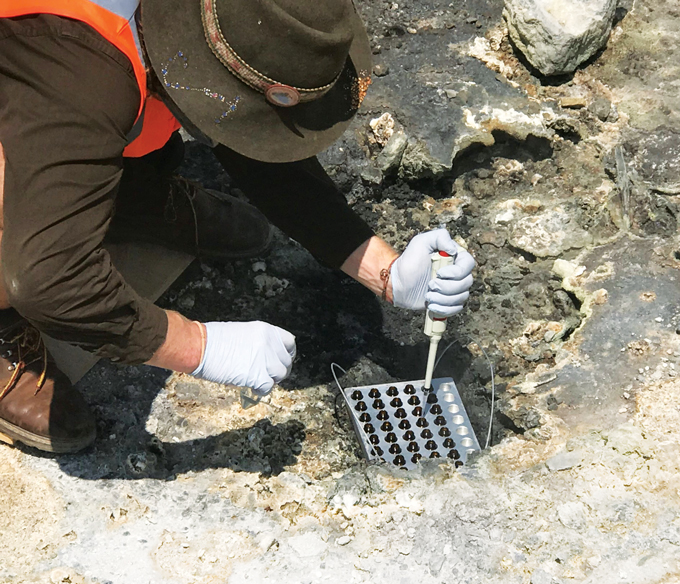
Damer stood the open vials in a metal block, roughly the size of two CD cases stacked together, and set the contraption into a near-boiling hydrothermal pool. To simulate the sometimes-wet, sometimes-dry burbling of the primordial Earth, Damer squirted acidic hot spring water into the vials, let them dry out and then repeated the wet-dry cycle several more times. When he brought the vials back to the lab, he found that they contained RNA-like strands that were 100 to 200 nucleotides long.
These results, reported in December 2019 in Astrobiology, indicate that complex molecules can form at hot springs, supporting the hypothesis that life on Earth may have developed in such an environment. In 2020, Damer returned to Hells Gate with Deamer and colleagues to confirm Damer’s results and do more wet-dry cycling studies.
Nicholas Hud, a chemist at Georgia Tech in Atlanta, studies the origins of life from a slightly different perspective: He explores how DNA and RNA nucleotides originated. He agrees that molecules are more likely to link together by condensation reactions on land, where wet-dry cycles can occur, than in the ocean. These reactions produce water; the formation of such a chemical bond isn’t energetically favorable when there’s already a lot of water around. “The best place to form that is in a hot, dry place,” Hud says. “The worst place to form it is in a wet, hot place.”
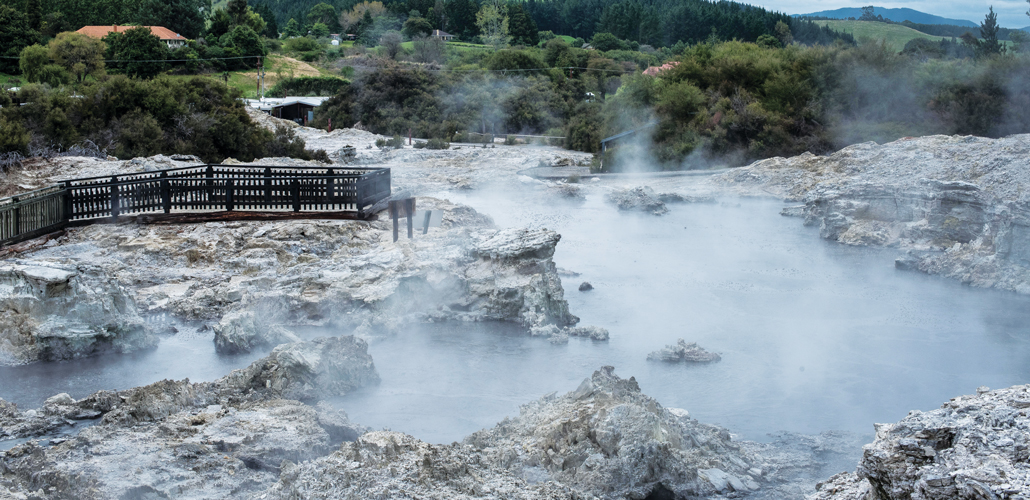
Underwater visions
Yet, wet, hot environs are just the place for life to originate, other evidence suggests. At hydrothermal vents on the deep, dark ocean floor, heated water spews into seawater that’s just a few degrees Celsius above freezing (SN: 7/23/16, p. 8).
In 2017, researchers found fossils in 3.77-billion-year-old rocks from Quebec that originated from the ancient ocean floor and had signs of hydrothermal activity (SN: 4/1/17, p. 6). The researchers claim that the distinct structures resemble those of microbes, suggesting that deep-sea environments may have supported some of the earliest life on Earth.
These environments can be extreme: Some vents belch dark plumes of water as hot as 400° C. However, if vents played a role in nurturing early forms of life, it likely happened at milder vents. For example, Lost City is a hydrothermal area in the middle of the Atlantic Ocean where the fluid streaming from vents ranges in temperature from 40° to 90° C. The region is named for dramatic limestone chimneys that rise as much as 60 meters above the seafloor.
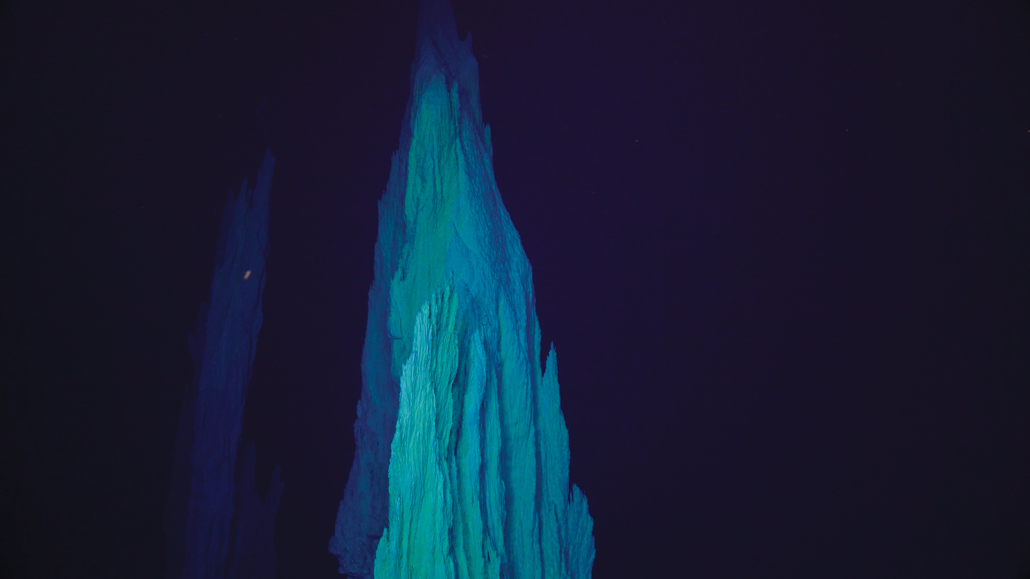
These spires are home to microbes that feed off the products of a chemical reaction known as serpentinization. “Hydrothermal vents are interesting because they are at the interface of water and rock,” says astrophysicist Laurie Barge of NASA’s Jet Propulsion Laboratory in Pasadena, Calif.
A chemical reaction between water and rock at sites like Lost City makes the water coming out of vents more alkaline than the water in the ocean, which has a higher concentration of positively charged hydrogen ions. The resulting gradient from alkaline to more acidic water is like the difference between the positive and negative ends of a battery and can serve as an energy source for chemical activity.
To study the conditions at underwater vents, Barge creates simulated environments in the lab that, she says, “can mimic what you see in the natural world.” To represent an ocean on early Earth, she fills an inverted glass bottle with an acidic mixture containing iron but no oxygen. One end of a plastic tube pokes through the narrow end of the bottle, connected to a steady supply of a basic, or alkaline, solution just like a vent.
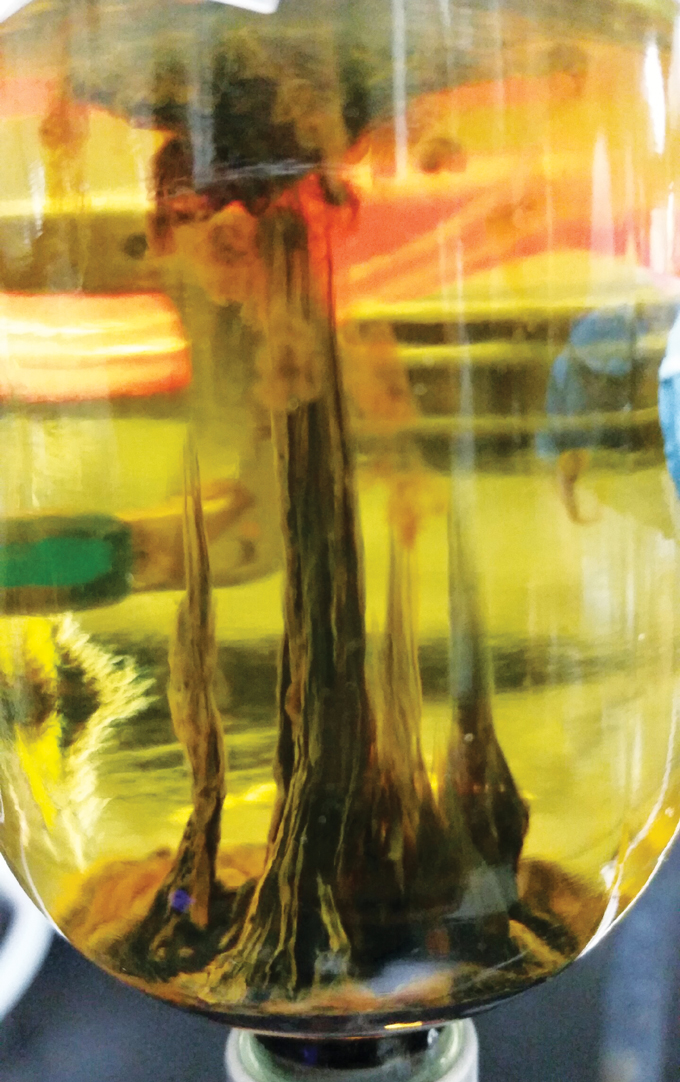
When Barge and colleagues injected an alkaline vent solution containing RNA nucleotides into an ocean-simulating bottle, individual RNA nucleotides linked up into short chains. These strands were only three or four nucleotides long, but the results suggest that the conditions at deep-sea vents could have supported reactions that led to the emergence of life on Earth, the researchers proposed in 2015 in Astrobiology.
Problems with both
To Deamer, there are big barriers to putting life’s pieces together near underwater vents: The vastness of the ocean would dilute molecules so they wouldn’t be concentrated enough to drive chemical reactions. Also, there are “no wet-dry cycles underwater.” In his view, repeated evaporation is needed to pull together enough molecules to bump into each other and react to form longer chains. Plus, unlike a hot spring’s freshwater, salty ocean water inhibits the formation of membranes and reactions that link together molecules, he says.
However, Deamer’s hot springs theory has its critics as well. DNA and RNA strands are composed of alternating phosphate and sugar molecules, but sugars “are profoundly unstable in hot spring environments,” says David Des Marais, an astrobiologist at NASA’s Ames Research Center.
And it may be too soon to rule out wet-dry cycles underwater. “You can have a little bit of water get stuck in a pore,” says Bill Brazelton, a marine microbiologist at the University of Utah in Salt Lake City. And then, because the serpentinization reaction at a vent uses up water in making other molecules, “you can have these cycles of dehydration inside a rock underneath the ocean.”
It may be impossible to nail down how life truly began on Earth: Most geologic records of what actually happened during Earth’s earliest days have long disappeared. There are numerous alternative hypotheses for where life began, beyond terrestrial hot springs and deep-sea vents. Recent research, for example, suggests that asteroid impacts could have sent superheated seawater into the crust to produce hydrothermal systems resembling hot springs (SN: 7/4/20, p. 10).
“I think we have to admit that there might be more than one little torturous path that might have been traversed in order for life to begin,” Des Marais says.
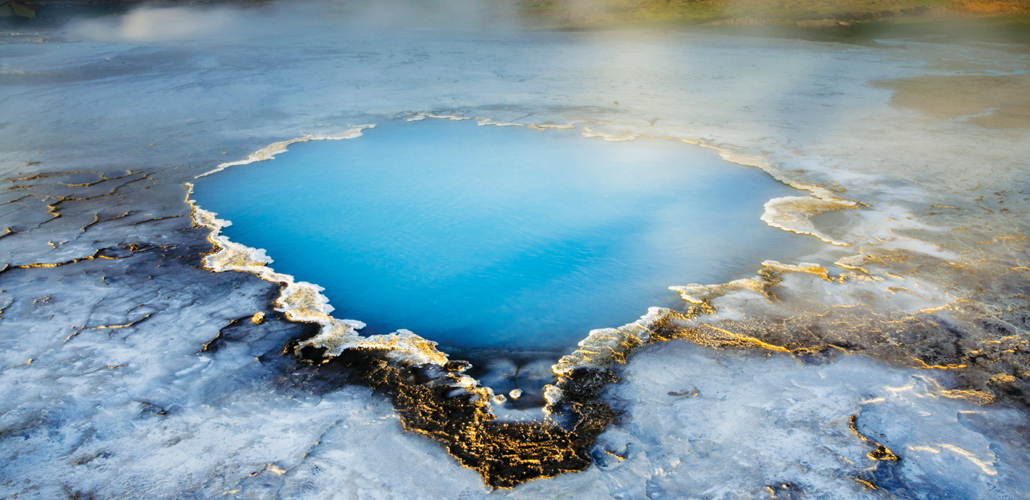
Life beyond Earth
Researchers are using what they’ve learned about how and where life may have originated on Earth to guide the search for biological signatures beyond our planet. There are several promising locales in our solar system.
“One of the things that NASA is really interested in knowing is whether or not there could be life in the subsurface oceans of the icy moons, like Europa and Enceladus,” says Batalha, of UC Santa Cruz. Scientists have evidence that the two moons, one orbiting Jupiter and the other, Saturn, have oceans of salty, liquid water beneath their icy shells (SN Online: 6/14/19).
These moons are intriguing because, along with liquid water, both have plumes of water erupting from their surfaces (SN: 6/9/18, p. 11), suggesting ongoing hydrothermal activity. NASA’s Cassini space probe even identified compounds containing carbon, nitrogen and oxygen within Enceladus’ plumes, some of the ingredients of amino acids, the building blocks of proteins. Europa and Enceladus fascinate astronomers because activity on their ocean floors may resemble the hydrothermal vents found on our own planet and may provide the chemical conditions to support life (SN: 4/18/15, p. 10).
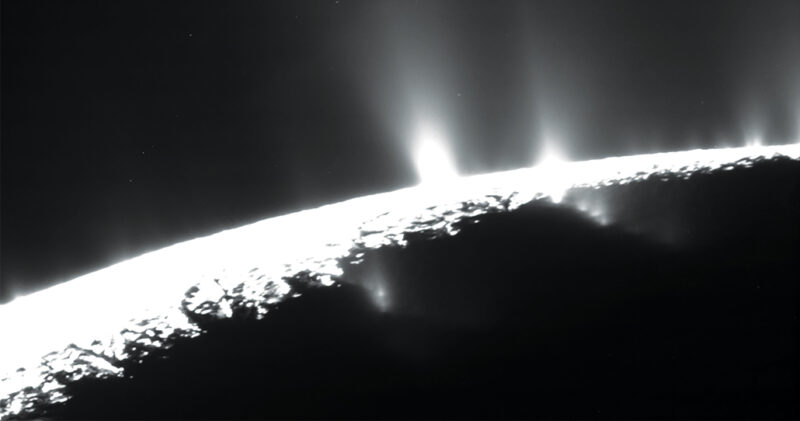
Icy moons may also promote condensation reactions. “Even if you were on an icy moon, you might have … freezing and thawing of ice,” Barge says. “So, I think it’s important to say, if wet-dry cycling is important, then we should look for any environment in the solar system that might be able to promote oscillating conditions of dehydration.”
But to find signs of past life, Damer and Deamer believe Mars is a more promising place to look. Mineral deposits indicate the presence of hot springs and hydrothermal activity in the planet’s past, which would have sustained the wetting and drying cycles that the two researchers see as crucial for condensation reactions to get life going.
Missions to the Red Planet are already under way. NASA’s Perseverance rover will be searching for signs of ancient life, such as telltale minerals in rock samples, at Mars’ Jezero crater when the mission lands in February 2021 (SN: 7/4/20 & 7/18/20, p. 30). Though at least 54.6 million kilometers separate them, Mars and Bumpass Hell may not be so different.